HIGH TEMPERATURE NANOINDENTATION
High temperature nanoindentation is a popular technique used to study the mechanical properties of materials at small length scales, with numerous applications in materials science, bioscience, coatings and additive manufacturing. Since mechanical properties are typically temperature-dependent, the development of high temperature nanoindentation has huge relevance to industrial applications where materials are used or processed at high temperatures.
The Development of Nanoindentation
Indentation tests encompass a range of techniques for determining the mechanical properties of materials. These typically follow the same format: indent a test material with a probe until an impression is formed, and characterize the mechanical properties of this material based on the size of the load, displacement of the indenter/sample and the geometry of the impression.
While macro-scale indentation techniques (such as the Vickers or Rockwell hardness tests) can give a useful relative measure of the hardness of different materials, more accurate measurements can only be achieved with small loads and indentations.1 This is taken to its logical conclusion with nanoindentation.
Nanoindentation is characterized by loads ranging from a few micronewtons to a few millinewtons distributed over areas ranging from a few square nanometers to a few square micrometers. The surface deformations produced by nanoindentation are typically so small that they cannot be resolved via optical microscopy.
Nanoindentation offers numerous advantages over macro-indentation. Indentations on this scale enable the mechanical properties of films, coatings and small samples to be measured without influence from the underlying substrate.2 In addition, nanoindentation can be used to examine microscale features or variations in material properties, such as the different constituent phases of complex microstructures.3
The first nanoindentation systems were developed in the 1970s, spurred on by increasing interest in microelectronics and biomaterials, which involved the study of thin films and small-volume samples.4 Nanoindentation has become a prevalent technique for material characterization, not least because many systems are capable of automated analysis and require only a clean, flat (and relatively small) sample to work with.5
With techniques developed in the early 1990s (the Oliver and Pharr analysis), these measurements can be directly obtained from a force-extension curve without the need to characterize the impression itself.6 A typical nanoindentation procedure can automatically produce statistically evaluated measurements of a wide range of mechanical properties, including hardness and elastic modulus.
High Temperature Nanoindentation
As with most mechanical properties, the hardness and elastic modulus measurements obtained via nanoindentation are temperature-dependent. In order to accurately characterize the mechanical behavior of engineering materials with high processing or operating temperatures, high temperature nanoindentation must be carried out.
High temperature nanoindentation involves controlled heating of the sample and indenter to high temperatures – temperatures up to 800 C are typically used. High temperature nanoindentation is frequently performed in a vacuum to prevent sample oxidation and other atmospheric effects.
High temperature nanoindentation presents a number of technical challenges, including temperature drift, sample oxidation and heat management. However, the development of high temperature nanoindentation systems like the Alemnis High Temperature Module (HTM) has enabled the widespread adoption of high temperature nanoindentation. This means that high temperature nanoindentation continues to increase in popularity and is now a standard method of probing temperature effects on material properties.
Applications of High Temperature Nanoindentation
High temperature nanoindentation provides a new avenue for fundamental materials research. The extremely localized nature of high temperature nanoindentation means that it can be used for in-depth investigations of the kinetic aspects of material behavior. High temperature nanoindentation has been successfully used to characterize a vast range of temperature-induced material effects. These include phase transitions in semiconductors like Si and Ge7, the crystallization behavior of bulk metallic glasses8, stress-strain of P91 steel9, and the atomic-level origins of plasticity in solids.10,11
High temperature nanoindentation also lends itself to use in conjunction with imaging techniques (such as SEM and TEM) or measurements of electrical measurements to create a broad understanding of material behavior at the nanoscale.12 Recent breakthroughs in these combined in-situ techniques allow real-time study of temperature effects on microstructure and the interplay between mechanical, thermal, and electrical effects at the nanoscale. Impact testing and multicycle indentation are also being used to investigate materials resistance to deformation at different temperatures.
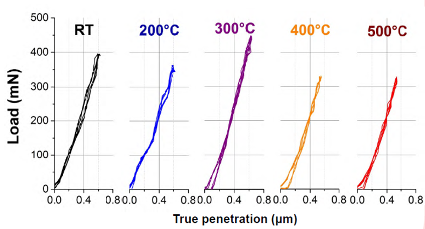
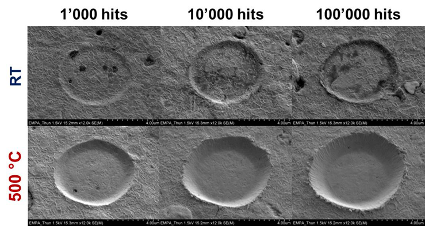
Load-displacement curves obtained between room temperature and 500°C for impact tests on a CrN coating with 10k impacts. Shown is an overlay of curves from the 20th to the 40th impact and residual imprints from room temperature and 500°C nano-impact tests (1k and 100k) using a diamond flat punch.
As nanoindentation testing uses small sample volumes, high temperature nanoindentation systems are well suited to rapid thermal cycling. This has been exploited, for example, in the investigation of thermo-mechanical fatigue of thin films, of particular interest in the electronics and semiconductor industries.5
In addition to compressive testing, high temperature nanoindentation systems developed by Alemnis also allow tensile testing. This enables high temperature tensile testing of microscale samples and features, such as those produced by focused ion beam or lithography.
Alemnis High Temperature Nanoindentation Systems
The Alemnis Standard Assembly (ASA) is the world’s most versatile indentation system, providing a modular platform for developing application-specific nanomechanical testing methods. Augmenting the ASA with one of four high temperature modules yields a robust, high-performance and cost-effective system for high temperature nanoindentation applications.
Alemnis high temperature modules support heating up to 200 C in air and up to 1000 C in vacuum. The system features three thermal sensors with < 1 C resolution and two independent temperature control loops for precise temperature setting and regulation. The result is a precisely controlled high temperature nanoindentation environment with a short temperature stabilization time.
Get in touch with Alemnis today to learn more about the capabilities of the ASA for high temperature nanoindentation applications.
References and Further Reading
- Fischer-Cripps, A. C. Introduction to Contact Mechanics. (Springer Science & Business Media, 2000).
- Fischer-Cripps, A. C. A review of analysis methods for sub-micron indentation testing. Vacuum 58, 569–585 (2000).
- Fischer-Cripps, A. C. The IBIS handbook of nanoindentation. (Fischer-Cripps Laboratories, 2009).
- Poon, B., Rittel, D. & Ravichandran, G. An analysis of nanoindentation in linearly elastic solids. International Journal of Solids and Structures 45, 6018–6033 (2008).
- Wheeler, J. M., Armstrong, D. E. J., Heinz, W. & Schwaiger, R. High temperature nanoindentation: The state of the art and future challenges. Current Opinion in Solid State and Materials Science 19, 354–366 (2015).
- Oliver, W. C. & Pharr, G. M. An improved technique for determining hardness and elastic modulus using load and displacement sensing indentation experiments. Journal of Materials Research 7, 1564–1583 (1992).
- Chavoshi, S. Z. & Xu, S. Temperature-dependent nanoindentation response of materials. 14.
- Nieh, T., Iwamoto, C., Ikuhara, Y., Lee, K. & Chung, Y. Comparative studies of crystallization of a bulk Zr–Al–Ti–Cu–Ni amorphous alloy. Intermetallics 12, 1183–1189 (2004).
- A. Ruiz Moreno, P. Hähner, F. Fumagallia, V. Haiblikova, M. Conte, N. Randall, Stress−strain curves and derived mechanical parameters of P91 steel from spherical nanoindentation at a range of temperatures, Materials & Design, 194, (2020) 108950
- Quantitative insight into dislocation nucleation from high-temperature nanoindentation experiments | Nature Materials. https://www.nature.com/articles/nmat1429.
- Choi, I.-C., Brandl, C. & Schwaiger, R. Thermally activated dislocation plasticity in body-centered cubic chromium studied by high-temperature nanoindentation. Acta Materialia 140, 107–115 (2017).
- Nili, H., Kalantar-zadeh, K., Bhaskaran, M. & Sriram, S. In situ nanoindentation: Probing nanoscale multifunctionality. Progress in Materials Science 58, 1–29 (2013).