COATINGS
The Alemnis Standard Assembly (ASA) has been used for a large number of studies on coatings in various forms. These can be standard coatings deposited onto reference substrates, coated 3D structures manufactured by additive manufacturing, coatings used in microelectronics and MEMS or micropillars of multilayers produced by focused ion beam (FIM) milling or selective lithography processes.
Example of a micropillar compression test on a micropillar built from a multilayer stack of different coatings
Modern hard coatings, especially those used in the cutting tool industry, have now become so hard in some cases, that conventional nanoindentation may not be the most appropriate method of testing them for mechanical properties such as hardness and elastic modulus. This is due to the fact that the hardness of the coating (for example Diamond-like Carbon layers with hardness of 30-40 GPa) is not so much less than that of the diamond indenter itself ( ̴ 70 – 120 GPa). In addition, many coating companies now want to know the properties of their thin films at service temperatures, which may be of the order of 500 – 900°C. At such high temperatures, the hardness of the diamond indenter will be significantly reduced and thus will deform dramatically, rendering the calibration of tip area function useless in calculating mechanical properties.
Recent studies have shown that testing small volumes of focused ion beam (FIB) milled coatings can be a much better way of measuring the mechanical properties of the material. These small volumes can be in the form of micropillars, tensile test bars and cantilever beams. In addition, as toughness is a key parameter for hard coating performance, microscale toughness tests can also be performed on beams, double-cantilevered bridges or double-cantilevered beams. However, the design of these test geometries requires prior knowledge of the yield strength to prevent plastic yielding from contributing to the apparent fracture energy.
Micropillar compression testing has now become commonplace with the ASA for characterizing hard coating properties and is particularly useful when probing the high temperature properties of coatings. The direct visualization of the deformation mechanism in-situ makes this a powerful and unique technique, where buckling, fracture or plastic deformation can be immediately observed and recorded.
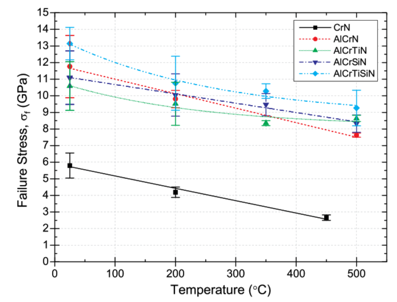
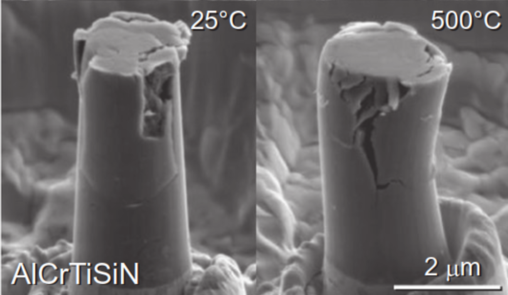
Example of failure stress data for 5 hard coating systems tested at 4 temperatures with example of AlCrTiSiN micropillars after testing at 25°C and 500°C (from Ref. 2)
In-situ scratch testing with the ASA is also being used to investigate failure mechanisms in coatings. In scratch testing, a diamond stylus is drawn across the sample under either constant or progressively increasing load. Elastic and/or plastic deformation occurs at specific points along the scratch path, such critical points being observed in-situ, by optical microscopy or by variations in acoustic emission and frictional force. In many cases, the scratch test has now become accepted as a versatile tool for assessing the mechanical integrity of a coated surface and has found application in many different fields of materials research. The driving forces for the failure of a coating-substrate system in the scratch test are a combination of elastic-plastic indentation stresses, frictional stresses and the residual internal stress present in the coating. The normal load at which failure occurs is called the critical load, Lc. When a progressive load scratch is performed, a number of consecutive coating failure events may be observed as the load is increased, the final event corresponding usually to total delamination. The critical load depends on coating adhesion, but also on several other parameters; some are directly related to the test itself (intrinsic parameters) whereas others are related to the coating-substrate combination (extrinsic parameters).
Examples of scratch tests on different coatings to better understand their failure mechanisms
Selected References
- L. Major, R. Major, M. Kot, J. M. Lackner, B. Major, Ex situ and in situ nanoscale wear mechanisms characterization of Zr/ZrxN tribological coatings, Wear 404 (2018) 82-91. https://doi.org/10.1016/j.wear.2018.03.011.
- J. M. Wheeler, R. Raghavan, V. Chawla, M. Morstein, J. Michler, Deformation of hard coatings at elevated temperatures, Surface & Coatings Technology 254 (2014) 382-387. https://doi.org/10.1016/j.surfcoat.2014.06.048.
- J. P. Best, G. Guillonneau, S. Grop, A. A. Taylor, D. Frey, Q. Longchamp, T. Schär, M. Morstein, J-M Breguet, J. Michler, High temperature impact testing of a thin hard coating using a novel high-frequency in situ micromechanical device, Surf. Coat. Tech. 333 (2018) 178-186. https://doi.org/10.1016/j.surfcoat.2017.10.072.
- J. P. Best, M. Polyakov, D. Shinde, M. H. Colliander, J. Wehrs, J. Michler, M. Morstein, Ni nanocluster composites for enhanced impact resistance of multilayered arc-PVD ceramic coatings, Surf. Coat. Tech. 354 (2018) 360-368. https://doi.org/10.1016/j.surfcoat.2018.07.102.
- F. Fanicchia, X. Maeder, J. Ast, A. A. Taylor, Y. Guo, M. N. Polyakov, J. Michler, D. A. Axinte, Residual stress and adhesion of thermal spray coatings: Microscopic view by solidification and crystallisation analysis in the epitaxial CoNiCrAlY single splat, Materials & Design 153 (2018) 36-46. https://doi.org/10.1016/j.matdes.2018.04.040.